Unlocking Quantum Advantage with Digital-Analog Quantum Computing (DAQC)
10.04.2025
Quantum computing is entering a transformative era, with rapid advancements bringing promising new paradigms into focus. One of the exciting and emerging approaches is Digital-Analog Quantum Computing (DAQC), a hybrid strategy uniquely positioned to harness quantum processor’s computational power to address more complex problems with existing commercial devices. Despite its great potential, DAQC has largely been overlooked by hardware providers of programmable quantum processors, with some successful but exceptional experimental confirmations (see Google’s Nature paper last year). This represents a critical gap in technology and provides an opportunity for companies to tap into DAQC’s immediate advantages.
The Essence of Digital-Analog Quantum Computing
DAQC merges two complementary quantum computing approaches: the precise, discrete control of digital quantum gates and the continuous multipartite or multimode dynamics of quantum processors. Digital quantum computing relies on the sequential application of precisely defined quantum gates. Companies such as IBM, Google, and IonQ are a few that make use of digital quantum algorithms. On the other hand, analog quantum computing leverages continuous quantum evolutions of bosonic and multiqubit operations, exemplified by quantum annealers such as D-Wave's processors and neutral-atom processors like those developed by QuEra and Pasqal. DAQC integrates these two strategies, offering greater flexibility, scalability, and potential for an early practical quantum advantage.
How DAQC Could Deliver Quantum Advantage
In practical terms, DAQC exploits the natural analog quantum dynamics of quantum processors in conjunction with digital quantum gates in an optimal manner, significantly simplifying complex computations. Via the smart encoding of complex problems into quantum hardware, DAQC can solve specific tasks with less algorithmic runtime, lower gate counts, minimal use of analog blocks, and reduced susceptibility to errors. This makes it especially appealing in the current noisy intermediate-scale quantum (NISQ) era, where error mitigation remains crucial. This clearly indicates that if quantum advantage can be achieved today with digital quantum computers, the systematic deploy of DAQC will make it even better.
For example, in superconducting circuits, the natural qubit-resonator couplings can be utilized to encode fermion-boson models natively, such as the Hubbard-Holstein model defined on a square lattice of size (l ×h) [DAQC of Fermion-Boson models with Superconductors], see Fig. (left). This model has relevance in material design and chemistry, where describing electron-phonon interactions accurately is essential for understanding superconductivity, charge-density waves, and other correlated electron phenomena.
Moreover, with Digital-Analog Counterdiabatic Quantum Optimization (DACQO) one can solve larger optimization problems at the quantum advantage level with a smaller gate count and less runtime using digital-analog encodings [DAQC for optimization with trapped ions], see Fig. (right). We have provided preliminary evidence of scaling up for optimization problems using DACQO with trapped ions, and we are now extending this approach to other hardware platforms, including superconducting circuits, neutral atoms, photonic, and semiconductor quantum processors. We have demonstrated that such digital-analog encodings, which combine native analog hardware interactions with digital gate operations, can lead to significant speedups in time-to-solution and improvements in solution quality.
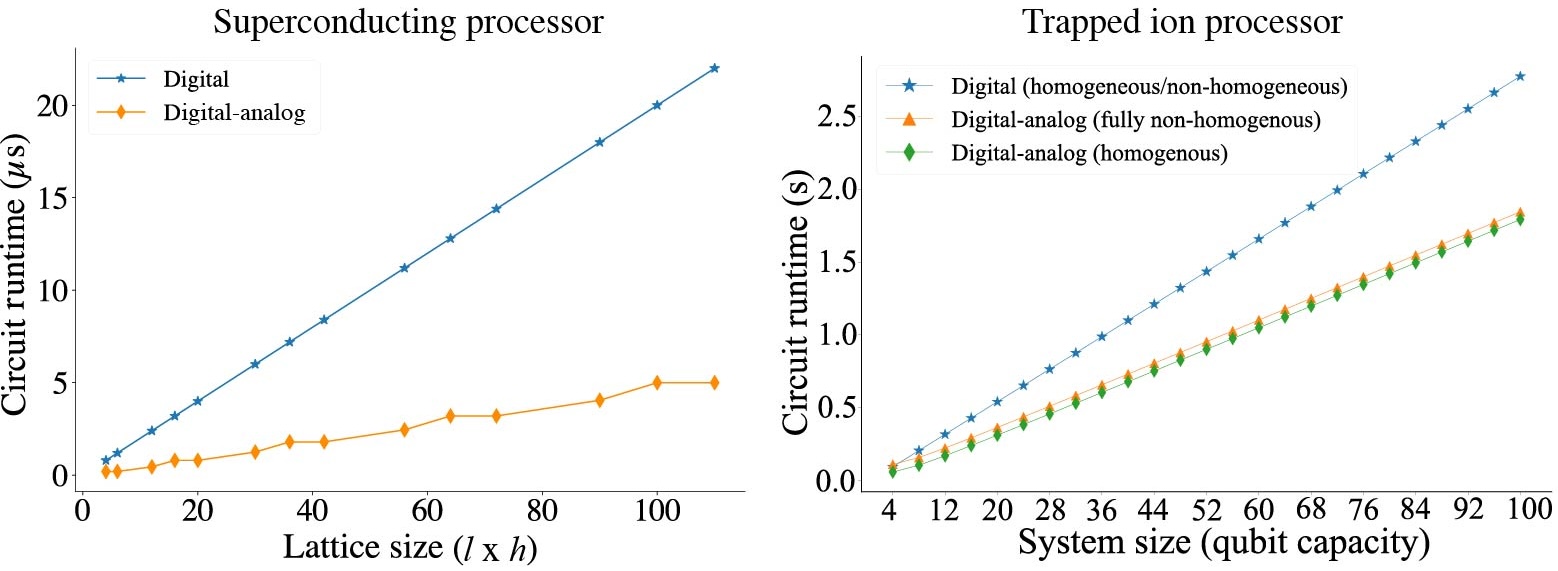
Real-World Applications of DAQC
Recent experiments done by Google [Google's Digital-Analog Quantum Processor] and QuEra [Quera's 100 Logical Qubits] have showcased the remarkable capabilities of leveraging analog interactions in superconducting circuits and neutral atoms for studying quantum phase transitions and advancing Quantum Error Correction, respectively. These breakthroughs highlight the potential of the Digital-Analog Quantum Computing (DAQC) paradigm across a range of high-impact applications:
- Drug Discovery: Accelerating molecular simulations to enable faster and more accurate screening of drug candidates.
- Optimization Problems: Efficiently tackling complex challenges in logistics, supply chains, and scheduling.
- Finance: Enhancing predictive modeling and risk analysis through quantum-accelerated computation.
- Materials: Simulating quantum many-body systems and exploring novel material phases with high precision, aiding the discovery of superconductors, catalysts, and quantum materials.
These achievements underscore DAQC's transformative potential—bridging the gap between theoretical quantum advantage and practical, real-world outcomes across industries
A Call to Hardware Providers
Despite its demonstrated benefits, DAQC remains underexplored by hardware providers of programmable quantum processors for demonstrating quantum advantage. To realize DAQC’s full potential, quantum hardware companies must recognize and invest in its unique capabilities. By doing so, they will not only facilitate the immediate practical application of quantum technologies but also position themselves at the forefront of quantum innovation. There is a potential to demonstrate quantum advantage using DAQC in Superconducting processors of Google, Rigetti and IQM, Neutral Atom processors of Quera and Pasqal, Trapped ion processors of AQT and eleQtron, Photonic processors of Quandela, and Spin-Qubit processor of Diraq.
Looking Ahead
The hybrid nature of DAQC can unlock immediate quantum advantage for wide classes of use cases and lay the groundwork for future quantum computing advancements. As quantum hardware matures, DAQC methodologies will seamlessly integrate and evolve, ensuring continual growth in computational capabilities.
DAQC offers quantum hardware providers a compelling opportunity to bridge the gap between theoretical promise and practical impact. Now is the moment to embrace digital-analog quantum computing and accelerate towards meaningful quantum advantage.
At Kipu Quantum, we are actively advancing the DAQC paradigm for quantum simulations of material properties, quantum chemistry, and complex optimization tasks. Our goal is to outperform classical techniques such as tensor network methods, Gurobi, simulated annealing, and CPLEX.